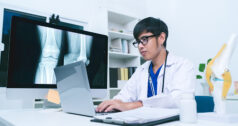
Smart implants are dynamic systems that collect and transmit clinical data in real time, offering valuable insights into postoperative recovery and implant performance. The devices monitor key variables such as pressure, strain, bone growth and healing progress, providing objective information about surgical outcomes and individualized patient care.
Orthopedic companies are taking notice and getting ahead of the innovation curve. Zimmer Biomet and Canary Medical led the way by developing smart technology for the Persona IQ knee implant, which measures and determines range of motion, step count, walking speed and other gait metrics. Intelligent Implants is developing the SmartFuse System, a PEEK and titanium cage that uses hermetically sealed electronics and an array of electrodes to accelerate bone growth in the lumbar spine.
The growing interest in smart implants goes beyond industry mainstays. University researchers and emerging startups are developing devices with the promise to advance digital ecosystems.
As the orthopedic industry focuses more on personalized care, companies are creating systems that translate clinical data into actionable insights. For example, smart sensors can detect implant instability or excessive stress, allowing healthcare providers to intervene early and tailor rehabilitation plans to each patient’s needs.
“The definition of a smart implant has evolved and now extends beyond simple sensing capabilities,” said Darryl D’Lima, M.D., Ph.D., who is involved in developing smart technologies as Director of the Shiley Center for Orthopaedic Research and Education (SCORE) at Scripps Clinic in San Diego. “A smart device needs to be implantable, collect data and provide intelligence — not just be a passive sensor. It should offer feedback to providers, enabling them to make informed patient care decisions.”
Designing a smart implant involves several critical components — sensors linked to a chip for data storage, an antenna for wireless communication and an enduring power supply — housed within the implant’s structure. Researchers are also focused on even more advanced applications, using metamaterials and specialized coatings to develop intelligent wireless, electronic-free devices.
It’s no simple task to pull off these levels of integration. Experts in the field possess the knowledge and sophisticated design skills that are needed to produce high-tech devices that are smarter than your average implant.
Material Selection: A Balancing Act
Designing a smart implant requires a careful selection of materials that offer biocompatibility and durability. Integrating sensors and communication systems adds another layer of complexity, necessitating additional considerations to maintain implant functionality. Dr. D’Lima pointed out that components like antennae must be insulated from the metallic parts of the implant to ensure they work properly.
“We usually choose materials, like titanium alloys, that are standard for implants,” Dr. D’Lima said. “But antennae need to be shielded from the titanium alloy. We borrowed a solution from cardiology and used a similar technology found in pacemakers.”
Integrating sensors into weight-bearing implants also poses unique challenges, as these devices must withstand high forces while maintaining functionality. “To measure force we use strain gauges, which detect how much the metal deforms under load,” Dr. D’Lima said.
“Deformity requires the metal to flex, which can potentially weaken the implant.”
To address this concern, his team conducts extensive mechanical testing, simulating millions of cycles to ensure the implant’s durability over its lifetime. They also use computer modeling to predict and optimize implant strength before production begins.
Qing Cao, Ph.D., Associate Professor of Materials Science and Engineering at the University of Illinois at Urbana-Champaign, also emphasized the importance of performance and safety in selecting materials for smart implants. His team is exploring innovative material combinations, such as polymers and single-crystalline silicon nanomembranes, to meet the specific needs of these devices.
The team has developed smart coatings for orthopedic surgical implants. These coatings monitor stress on the implants, providing early detection of potential failures and combatting harmful bacteria that may cause infections.
To construct the outer surface of the coating’s nanostructured foil, the team fabricated high-density arrays of nanopillars with optimized geometries that mimic the natural structures of cicada and dragonfly wings.
“By placing sensors on the surface of implants, we avoid the need to alter its core structure, which reduces the risk of compromising its strength,” Dr. Cao said.
The research team published a study in the journal Science Advances that demonstrated that the technology was successful in preventing infection in live mice and mapping implant strain in the spines of sheep.
They will begin testing the smart coating on larger animal models with skeletal properties similar to humans. “If everything goes well, we will then test the technology in humans,” Dr. Cao said. “It will take several years before we can complete these experiments and meet FDA’s regulatory requirements. Our current target is to make the coating available for clinical use by 2029.”
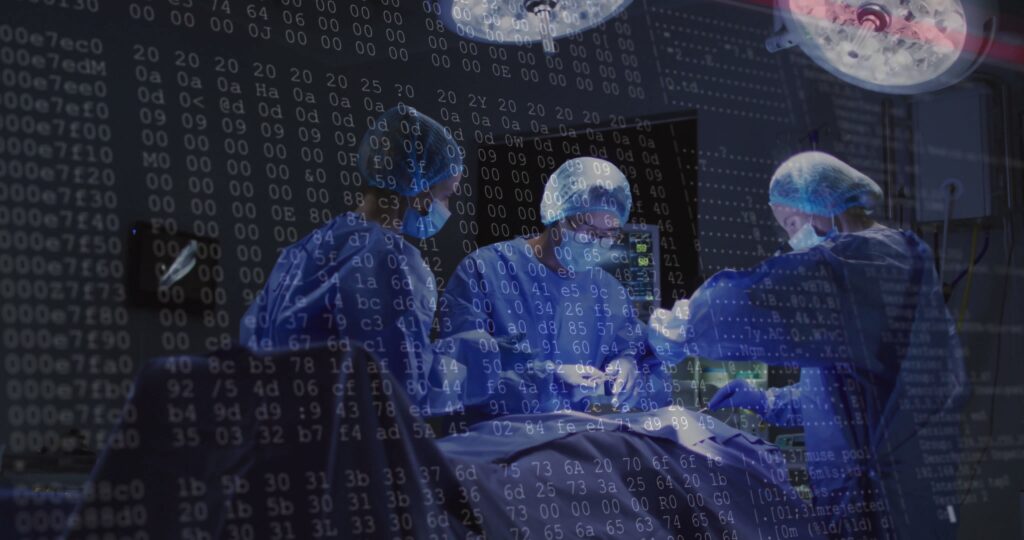
Smart implant technology feeds the digital ecosystems that drive the push for personalized patient care.
Capturing Reliable Data
Long-term sensor accuracy is crucial for smart implants, as the human body presents an environment where sensors can easily malfunction or provide inaccurate data. That’s why it’s important to continuously calibrate and test sensors even after implants are deployed.
“Sensors can’t be allowed to drift or degrade,” Dr. D’Lima said. “We perform regular checks to ensure the sensors remain accurate. Zero is an easy calibration after implantation, so we often take the weight off or unload the implant to see if the sensor returns to zero force. It’s an effective way to check reliability and accuracy.”
Dr. D’Lima also noted that issues such as cracking or separation of the bonding between sensor and implant can lead to drift or changes in sensor readings. His team uses advanced techniques, such as force plate calibration, to validate the implant’s performance under real-world conditions, ensuring its long-term reliability.
“You want the implant and its sensors to last the lifetime of the patient,” he explained.
Clinical trials play a crucial role in evaluating the effectiveness and safety of smart implants before they can be widely adopted. Dr. D’Lima and his team have been conducting trials that focus on smart knee implants, testing the long-term reliability of strain gauges and other sensors in monitoring patient recovery. These trials are designed to simulate the real-world conditions that patients face, such as varying levels of activity and different rehab protocols.
“We partnered with several rehabilitation centers to gather data from patients with smart implants, allowing us to refine our technology based on real-time feedback,” Dr. D’Lima said. “The information is being used to track activities of daily living, design rehab programs and even provide insights on the safety of recreational activities for recovering patients.”
This approach not only tests the durability of the implants but also provides invaluable data that feeds back into the design and development cycle, ensuring that future implant iterations are more accurate and reliable.
Dr. Cao’s research focuses on maintaining sensor accuracy while minimizing structural compromise. He explained that sensor placement is a key challenge. Sensors can be mounted on the implant’s surface or housed within a cavity. Both options have drawbacks.
“Sensors on the surface of implants, which are typically not flat, need to be mechanically flexible,” he said. “Flexible strain sensors that are based on simple metal lines formed on flexible plastic substrate have poor sensitivity, compromising their accuracy.”
According to Dr. Cao, strain sensors built on single crystalline semiconductors can offer up to 100 times higher sensitivity. However, he noted, single crystalline semiconductors like silicon that are used to make high-performance computer chips are typically rigid.
“You can’t house them on the curved surface of implants. You could put them inside the implant, which can create more sensitive data readings,” he said. “But that can compromise the implant’s structural integrity, and that’s the last thing you want.”
According to Dr. Cao, the answer is to use ultra-thin, flexible sensors. “We’re developing high-sensitivity sensors based on single-crystalline silicon membranes that can be wrapped around the implant’s surface rather than embedded inside,” he said.
Sensors that wrap around the surface of the implant and demonstrate high performance provided by single-crystalline semiconductors combine the important elements of flexibility and function.
“The ultimate goal is to make conventional orthopedic implants smart without compromising their mechanical structures,” Dr. Cao said.
Providing Reliable Power
Powering sensors and communication systems within smart implants is a significant technical challenge, especially in weight-bearing areas like the knee or spine. Dr. D’Lima’s lab has explored various methods, including electromagnetic induction and near-field communication (NFC) technologies.
“Our early designs relied on external coils,” he said. “Since then, we’ve been able to provide onboard power storage, so the latest generations now use rechargeable batteries that can last for a week at a time.”
NFC’s other advantage is that it can support data transfer and charging at the same time, so only one antenna is needed in the implant’s design.
Dr. Cao’s research supports these advancements. His team is testing wireless communication systems to power and communicate with the smart implant, focusing on optimizing energy efficiency while maintaining reliable data transmission. “In the case of spinal implants, which are close to the skin, we can use short-range NFC that requires less power,” he said.
While most smart implant designs require some form of external charging, researchers are exploring ways to harness the body’s natural energy. Dr. D’Lima highlighted ongoing research at SUNY Binghamton, where a triboelectric energy harvester has been developed. This device uses the frictional energy generated by the implant as the patient walks to power the electronics.
Researchers at the University of Pittsburgh Swanson School of Engineering have developed a 3D-printed smart metamaterial spine implant. The device leverages highly sophisticated technologies to double as a self-powered sensor that monitors bone healing in the spine.
“That’s the convergence the field is hoping for,” Dr. D’Lima said. “As the electronics continue to develop to run on low power, we won’t need external coils or batteries.”
Future Potential
As technology advances, the potential for smart implants expands beyond traditional orthopedic applications. Dr. D’Lima envisions a future in which implants not only monitor mechanical forces, but also integrate biochemical sensors to detect infection or other metabolic changes.
“Imagine a smart implant that could monitor glucose levels or detect early signs of cancer,” he said. “This could transform how we manage chronic conditions.”
However, regulatory hurdles remain a significant challenge for smart implant developers. Dr. D’Lima pointed out that FDA has not yet established standardized protocols for these devices, making the approval process complex and unpredictable.
“It’s a moving target,” he said. “We’re involving FDA early in our development process in the hope that we can help the agency monitor future implants more effectively.”
This collaborative approach aims to set a precedent for regulatory standards, ensuring that smart implants meet stringent safety requirements.
Dr. Cao’s team is currently preparing for large animal trials to validate their technology before seeking regulatory approval. “We need to demonstrate that our sensors provide meaningful and actionable data in comparison to the current gold standard of post-op monitoring that’s based on radiology imaging,” he said.
Dr. Cao also highlighted the role of machine learning in enhancing smart implant functionality. His team is integrating these capabilities to create a comprehensive system that can monitor and respond to various physiological changes.
“By collecting and analyzing large amounts of data over time, we can make more accurate predictions about patient outcomes,” he said. “This could lead to more personalized and effective treatments.”
Looking ahead, Dr. Cao emphasized the importance of continued innovation in smart implant technology.
“We need to keep exploring novel materials, advanced electronics and communication systems and machine-learning-assisted data analysis, while ensuring patient safety and privacy,” he said. “It’s a delicate balance, but the possibilities are exciting.”
KK
Kendal Kloiber is a contributing editor.