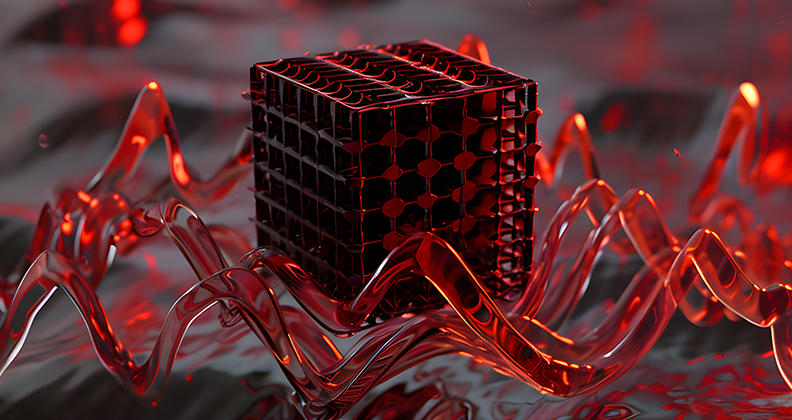
Researchers at the University of Pittsburgh have developed metamaterial interbody fusion cages with the stiffness to stabilize vertebrae movement, the flexibility for load-sharing and the porosity to support bone on-growth and in-growth.
It’s the holy grail of spine implants, according to Amir Alavi, Ph.D., an Assistant Professor in the Department of Civil and Environmental Engineering at the University of Pittsburgh’s Swanson School of Engineering.
His research team received funding from the National Institutes of Health (NIH) for the first-ever testing of metamaterial orthopedic implants. They’re developing spinal fusion cages that incorporate stabilizing, load-sharing and bone ingrowth functionalities in a seamless design.
Dr. Alavi pointed to the inherent limitations of titanium and specific polymers like PEEK, which are commonly used materials in fusion cages because of their biocompatibility, strength and durability.
“While one might assume that the high rigidity of metal implants is desirable, it actually can lead to extreme compression, delayed bone healing and even catastrophic destruction of the host bone,” he explained. “There are also major concerns regarding the bone integration of PEEK cages.”
Metamaterials are more advanced than traditional elements, alloys and other materials because they can be designed to provide a wide range of desired mechanical properties, Dr. Alavi noted.
“We firmly believe that our metamaterial approach to designing interbody fusion cages is the most viable strategy for seamlessly integrating essential features into a single device,” he said.
Advanced Form and Function
Using metamaterials for implant systems provides a vast design space because they can be fabricated using various biocompatible materials, Dr. Alavi noted.
“Once we design the proper single- or multi-material unit cell with the desired functionality — matching it to a specific patient’s bone density, for example — we can assemble it into various shapes to form any structure,” he said.
Scalability is one of the most outstanding features of metamaterials, according to Dr. Alavi, who noted they can be shaped and 3D-printed to precisely fit an individual patient’s anatomy.
Dr. Alavi said the tailored level of mechanical tuneability afforded by metamaterial implants is obtained through the systematic arrangement of “rationally designed” unit cells. “This approach enables us to utilize various biocompatible materials, or combinations of materials, to form a composite, multi-material lattice,” he said.
Smart Design Elements
Dr. Alavi’s team recently demonstrated mechanical tunability — tunable stiffness and strength, and load-sharing functionality — of a multi-material metamaterial spinal fusion cage. He said combining various biocompatible materials in constructing the metamaterial cage lattice creates the possibility of creating intelligent wireless, electronic-free devices.
“To underscore this potential, we have engineered the composite lattice to function as a sensing and energy harvesting medium,” Dr. Alavi explained. “The cage can convert the mechanical forces caused by spine micro-motions into electrical signals. It essentially serves as a mechanically tunable device with an intrinsic built-in nanogenerator.”
Testing showed that the electrical signals generated by nanogenerator metamaterial cages are proportional to the spinal forces and can be used for in vitro monitoring of spinal fusion in human cadaver models.
“The electrical signals can be used for electrical stimulation for accelerating bone growth or wireless transmission of the sensed signal,” Dr. Alavi said. “A groundbreaking discovery in our recent study was that the force-induced electric field generated by our metamaterial implants, even with a power output as low as 0.1 pW, can propagate through porcine tissue and subsequently be wirelessly detected using electrodes.”
Integrating these advanced functionalities into the structure of a fusion cage cannot be achieved with existing technology, according to Dr. Alavi.
“For these reasons, we believe that the metamaterial implants represent the holy grail in orthopedic innovation,” he said. “Serving as all-in-one wireless force sensing units, they incorporate functions for power generation, continuous force sensing and wireless transmission — without relying on external antennas, power sources or telemetry systems.”
Continuous Improvement
Metamaterial fusion cages can improve patient outcomes in several ways, according to Dr. Alavi.
First, as mechanical devices with tunable stiffness, strength and energy absorption, they can be designed with local compliance and reduced stiffness to facilitate more consistent load-sharing throughout the spine’s full range of motion, from flexion to extension.
“This is clinically significant as load-sharing through the interbody space stimulates bone formation and maturation, potentially leading to better outcomes,” Dr. Alavi said. “Additionally, their morphological parameters, such as void shape, size and permeability, can be optimized to support bone growth.”
Second, the electrical signals generated from spine micro-motions can be utilized for the wireless monitoring of bone healing progress and potentially for electrical stimulation to accelerate bone growth. “Continuously monitoring the spinal fusion progress can provide important information about the stability and load-sharing at the intervertebral disc space level,” Dr. Alavi said.
Fabricating intelligent metamaterial implants, which are multi-layered and multi-material systems, presents challenges compared to single-base material metamaterial implants.
“While nanofabrication technologies can readily be employed, rapid prototyping with multiple materials using 3D printing is challenging,” Dr. Alavi said. “PolyJet 3D printing stands out as the preferred option for metamaterial implants, although FDM printers could work, depending on the resolution requirements.”
Dr. Alavi’s team has packaged and validated metamaterial fusion cages for wireless monitoring of spinal fusion in human cadaver models. As part of the ongoing NIH project, they are investigating the mechanical performance of metamaterial fusion cages in vivo using ovine cervical spine models.
“This marks a historic milestone because it will be the first in vivo trial of metamaterial studies to study other functionalities of the implants,” he said.
DC
Dan Cook is a Senior Editor at ORTHOWORLD. He develops content focused on important industry trends, top thought leaders and innovative technologies.