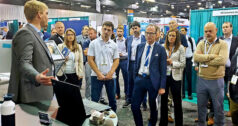
The perfect orthopedic implant doesn’t exist. Or does it? Multi-metal printing allows R&D engineers to concoct a single device made with the best ingredients of select metals. A dash of cobalt chrome’s wear-resistant articulating surface here, a sprinkle of titanium’s biocompatibility there and voila: a made-to-order implant designed for optimal performance.
Amnovis, a contract manufacturing and engineering firm, is at the forefront of the multi-metal printing movement. The company uses a drum-based printing platform in which powder from two different materials is sucked onto the drums, which roll around build plates while selectively depositing the powder in specific positions.
Two drums are currently operational in the Amnovis facility. A team of operators controls the speed at which the drums work and the different powders that are deposited to produce fully completed multi-metal parts. Voxel technology allows Amnovis to control the deposition of the powder in three dimensions. The process achieves high marks in precision and accuracy.
Multi-metal printing is one of many developments in the additive manufacturing space that highlights 3D printing’s promising future in orthopedics.
“Engineers who design and develop new implants need a mentality shift and an awareness of the kind of design freedom multi-metal printing offers,” said Maxime Salingros, Technical Sales Engineer at Amnovis. “They need to consider the possibility of making implants with various 3D-printed metals. If they don’t know about it, they can’t make it.”
Combined Benefits
So, what is there to know about the possibilities of the multi-metal printing process? Consider a 3D-printed femoral implant that’s typically made of cobalt chrome, including the post and internal structures. Cobalt chrome effectively prevents wear and tear, but is not the best material to promote bone ingrowth. Multi-metal printing allows product engineers to design an implant with the durability of cobalt chrome on the articulating surfaces and inside structures with the porosity of titanium.
Patella locking plates offer another example. The devices are currently produced with titanium grade 2 or grade 4.
“However, the plates are usually very thick and relatively inconvenient to use due to the lower strength of those titanium grades compared to titanium alloy,” Salingros said. “Multi-metal printing could be used to include the strength of titanium alloy while keeping the functionality of titanium on the outside surface so the plates can be bent to fit the patient’s anatomy.”
If it all sounds too good to be true, it might be. At least for now.
“Much focus has been placed on developing the hardware needed for multi-metal printing, but many challenges remain in optimizing the technology,” Salingros said. “Most of the potential metal combinations — copper alloys, aluminum steels — have been studied for industrial applications. We’re unaware of combinations in development that would be suited for medical devices.”
Products printed with combined metals in the industrial space have resulted in cracks, pores and defects forming at the interface because the chemical and metallurgical reactions are highly complex. “We need to develop this technology further to avoid similar mechanical failure,” Salingros said.
Practical challenges also remain in scaling multi-metal printing, including the high cost of the required hardware — there are few available modules that can print various metals.
The speed at which the drums operate is still relatively slow compared to laser powder bed fusion printing methods. It’s also difficult to prevent cross-contamination of the metal powders without working with new batches for each cycle. The availability of suitable CAD/CAM software is also a concern, according to Salingros, who said it’s not developed enough to evolve and work as rapidly as it should for this application.
Amnovis continues to develop the multi-metal printing process and aims to achieve technology readiness over the next four years, according to Salingros.
Bend But Don’t Break
Contract manufacturer Croom Medical wants to leverage additive manufacturing into improved patient outcomes, primarily by closing the gap between the dissimilarity in the consistency of materials used to make implants and actual bone. “The phenomenon of stress shielding can lead to bone loss and the aseptic loosening of implants,” said Shane Keaveney, R&D Manager at Croom Medical.
Additive manufacturing allows orthopedic manufacturing to print complex shapes and interesting geometries and presents the potential for patient-specific implants. “We wanted to figure out how to combine the benefits of 3D printing and tantalum,” Keaveney said.
Croom Medical recognizes that tantalum can be used to produce implants that promote better osteointegration and biocompatibility. The material also has mechanical properties that make it well-suited to produce effective and versatile implants.
Keaveney said tantalum has a large zone of plastic deformation, which makes it a tough but malleable material. It’s also already been used in medical devices and has been proven to have superior osteointegration and biocompatibility. Its high melting temperature of around 3,000°C makes it a chemically stable material that performs well in terms of thermal conductivity.
The oxygen sensitivity of tantalum is also a key consideration. “The powder’s oxygen content dramatically affects its performance in additive manufacturing,” Keaveney said.
Tantalum can be used to make intricate structures with good consistency and quality, according to Keaveney. He said Croom Medical is currently putting the material through compression tests to understand the full scope of its potential in additively manufactured implants.
The tests have shown that tantalum did not suffer strut failures after the complete deformation of its core structure. In contrast, the literature has shown that stress tests cause 45-degree shear and brittle-type failure of struts in titanium.
“We haven’t seen results like that in our tantalum samples,” Keaveney said. “Tantalum with strut sizes from 150 to 300 microns showed very soft failure, which can have a positive impact on patient outcomes. There’s a potential to use this feature as an advantage.”
For example, pressure on a tantalum implant or an unexpected loading on a porous coating won’t result in brittle failure or the release of debris, according to Keaveney. He pointed out that surgeons who want to match an implant’s porous structure to the topography of bone can allow the implant to deform because they know the material won’t break, crack or fail.
“The effects of stress shielding are also reduced on tantalum, so it can more closely match the modulus of bone,” Keaveney said.
He also referenced the stability of tantalum powder. “We observed minimal change in the powder’s consistency over a cycle of builds after 30% of a lot had been processed,” he explained. “The powder’s oxygen content picked up only 30 to 35 parts per million. That’s a very small amount.”
Croom Medical partnered with Global Advanced Metals (GAM) to develop tantalum’s additive manufacturing capabilities. GAM is a manufacturer of high-purity tantalum powder and has worked for many years to develop its product for 3D printing.
“The quality of the powder is key in additive manufacturing,” Keaveney said. “Working directly with GMA allows us to tune the powder and optimize the supply chain process. We can scale the production of printed devices and make them available to our customers.”
Partnering with a supplier allows Croom Medical to tune the tantalum powder to optimize its printing performance. The partnership also reduces the overall costs of the powder supply.
“We send back the leftover amount, which GAM recycles at the appropriate stage in the production process,” Keaveney said. “Although tantalum powder is more expensive than other metals, the GAM partnership lessens the cost burden for our customers.”
The two companies intend to lower the barrier to entry for orthopedic companies to use tantalum within their devices. “We’re innovating and pushing past what’s currently possible in additive manufacturing,” Keaveney said. We want to find partners that want to use tantalum technology in their devices and benefit from its features.”
Croom Medical will conduct animal testing to compare its in-house results with tantalum to the reported literature. The company is also examining current constructs to determine if the properties of tantalum can be combined with titanium to optimize the form and function of implants.
“How can we print tantalum onto titanium? What’s that interface like? How will the production work?” Keaveney asked. “Those are key research questions for us.”
Building Better Bone
Adam Jakus, Ph.D., Co-Founder and Chief Technology Officer of Dimension Inx, first became interested in the capabilities of additive manufacturing nearly a decade ago while working with Ramille Shah, Ph.D., in a Northwestern University lab. The two colleagues quickly realized that advanced 3D printing was ideally suited to produce complex structures.
They also had a background in materials and tissue engineering and were intrigued by the possibility of producing a 3D-printed substance that would eventually turn into the bone or tissue in which it was implanted.
Their curiosity led to the development of a 3D printing platform that produced Hyperelastic Bone, a combination of hydroxyapatite and a biocompatible, biodegradable polymer. The material is robust and porous at the nano, micro and macro levels. This design allows blood vessels to infiltrate the structure to initiate tissue regeneration.
“Porosity is huge when it comes to tissue regeneration because you want cells and blood vessels to infiltrate the scaffold,” Dr. Shah said. “Our 3D structure has different levels of porosity that are advantageous for its physical and biological properties.”
Cells can sense the hydroxyapatite in the Hyperelastic Bone and respond to its bioactivity, according to Dr. Shah “When you put stem cells on our scaffolds, they turn into bone cells and start to up-regulate their expression of bone-specific genes,” she said. “This is in the absence of any other osteo-inducing substances. It’s just the interaction between the cells and the material itself.”
Hydroxyapatite induces bone regeneration, but the substance is hard and brittle and difficult to shape. Drs. Shah and Jakus designed and printed the structure of Hyperelastic Bone in a way that maintains its elasticity. It was designed to have the excellent handling properties of a polymer and the bioactive properties of ceramic.
“About 90% of the material contains ceramic — more than what’s in natural bone — but the microstructure includes porosity and the macroscopic material has some flex to it,” Dr. Jakus said. “Microscopically, the cells of the body recognize it as a ceramic material.”
The off-the-shelf product comes in simple geometric shapes, but because it’s so malleable, surgeons can alter the form to fit the patient’s defect, which Dr. Jakus said is typically difficult to accomplish with metals and alloys.
“Bones are brittle and they break, so you’d think a material with so much calcium phosphate in it would be brittle,” Dr. Jakus said. “But what makes the formulation of Hyperelastic Bone unique is that, despite being mostly ceramic, it’s not brittle. It can be cut and rolled in a thin sheet form. It’s very easy to handle and surgically implement.
“Surgeons want products that are easy to work with — that are easy to form into irregular shapes and place in defects,” Dr. Jakus continued. “That’s often a forgotten element in new product design.”
Hyperelastic Bone is being manufactured and commercialized through Dimension Inx and has received FDA clearance for use as a 3D-printed off-the-shelf maxillofacial bone graft. “The product is an example of how these types of advanced technologies are no longer just scientific curiosities and are now starting to be manufactured and enter the market,” Dr. Jakus said.
Dimension Inx’s patented materials platform combines 3D-painting materials and a manufacturing system that allows for the rapid printing of numerous kinds of biomaterials. The 3D paints are printed at room temperature and dry nearly immediately without the need for sintering, curing or cross-linking.
“The particles that we use determine the functionality of the 3D paints,” Dr. Shah said. “To have multifunction within one device is what allows us to target certain areas in tissue repair and regeneration.”
The technology is indicative of huge gains being made in additive manufacturing. “Look to smaller startup companies to get a sense of what’s coming next in the space,” Dr. Jakus said. “That’s where innovation is happening.”
DC
Dan Cook is a Senior Editor at ORTHOWORLD. He develops content focused on important industry trends, top thought leaders and innovative technologies.